Active Matter
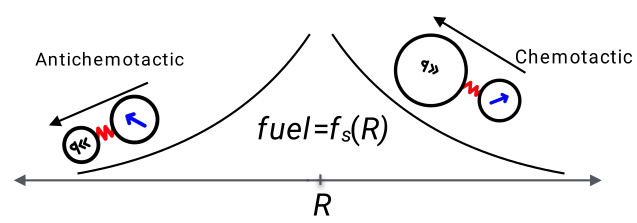
Active particles with their characteristic feature of self-propulsion are regarded as the simplest models for motility in living systems [1]. The accumulation of active particles in low activity regions has led to the general belief that chemotaxis requires additional features and at least a minimal ability to process information and to control motion [2]. Active particles can exhibit interesting transient behaviour in fuel gradients, showing a tendency to move towards the high activity regions, called pseudochemotaxis [3]. However, activity does not lead to an advantage in the search for fuel sources compared to passive diffusion on long timescales [4].
Recently, we showed that self-propelled particles display chemotaxis and move into re- gions of higher activity if the particles perform work on passive objects, or cargo, to which they are bound [5]. The origin of this cooperative chemotaxis is the exploration of the activity gradient by the active particle when bound to a load, resulting in an average excess force on the load in the direction of higher activity. Using a new theoretical model, we captured the most relevant features of these active-passive dimers, and accurately predicted the crossover between an- tichemotactic and chemotactic behaviour. We further showed that merely connecting active particles to chains is sufficient to obtain the crossover from antichemotaxis to chemotaxis with increasing chain length. The observed transition is of significance to protoforms of life, enabling them to locate a source of nutrients even in the absence of any supporting sensomotoric apparatus. We are currently working on the stochastic thermodynamics of active-passive dimers.
References:
[1] M. E. Cates and J. Tailleur, “When are active brownian particles and run-and-tumble particles equivalent? consequences for motility-induced phase separation,” EPL (Europhysics Letters), vol. 101, no. 2, p. 20010, 2013.
[2] B. Liebchen and H. Loewen, “Synthetic chemotaxis and collective behavior in active matter,” Accounts of chemical research, vol. 51, no. 12, pp. 2982–2990, 2018.
[3] H. D. Vuijk, A. Sharma, D. Mondal, J.-U. Sommer, H. Merlitz, "Pseudochemotaxis in inhomogeneous active Brownian systems," Phys. Rev. E, 97, 042612, 2018.
[4] H. Merlitz, H. D. Vuijk, R. Wittmann, A. Sharma, J.-U. Sommer, "Pseudochemotaxis of particles competing for food," Plos One, 15, e0230873, 2020.
[5] H. D. Vuijk, H. Merlitz, M. Lang, A. Sharma, J.-U. Sommer, "Chemotaxis of cargo-carrying self-propelled particles," Phys. Rev. Lett., 126, 208102, 2021.